
RETURN to Periodic Table
Boron is the 5th element on the periodic table because it has 5 protons in its nucleus. The protons will attract 5 electrons to surround the nucleus in order to form a neutral atom. With 5 protons and 6 neutrons, most boron atoms have an atomic mass of 11 amu.
Electron Shell
With five electrons, boron is the first atom to contain electrons that are in a p-orbital. This is not a sphere-shaped harmonic, and so a single electron in a p-orbital cannot find a symmetrical arrangement around a sphere by itself. Boron therefore cannot simply add its p-electron on top of the same (2s2) configuration that beryllium has, as shown here, because it would not be stable:

The asymmetry therefore causes the two electrons in the 2s orbital to uncouple from their di-electron state and form a tri-electron state with the single p-electron. This is called hybridization.
Electron Orbital Hybridization
Through hybridization, the three 2nd shell electrons now have equal energy (degenerate) and they can achieve maximum stability by assuming a trigonal planar arrangement around the core electron shell. Their mutual repulsion is minimized by having them as far from one another as they can get. This geometry is called an ‘sp2-hybridization’ because it involves 1 s-orbital box and 2 p-orbital boxes to create 3-directional symmetry. (The large wireframe indicates the boundary of the n=2 shell.)
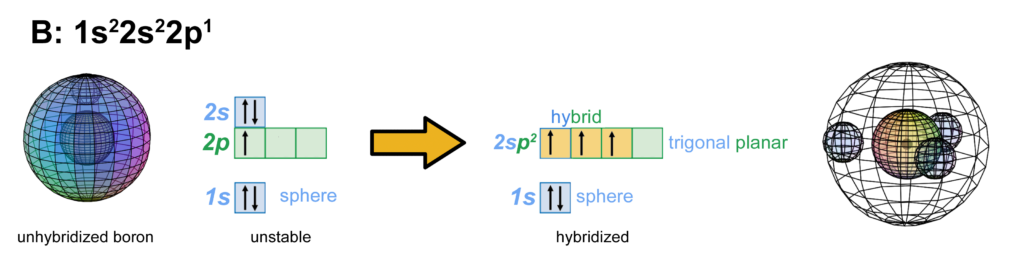
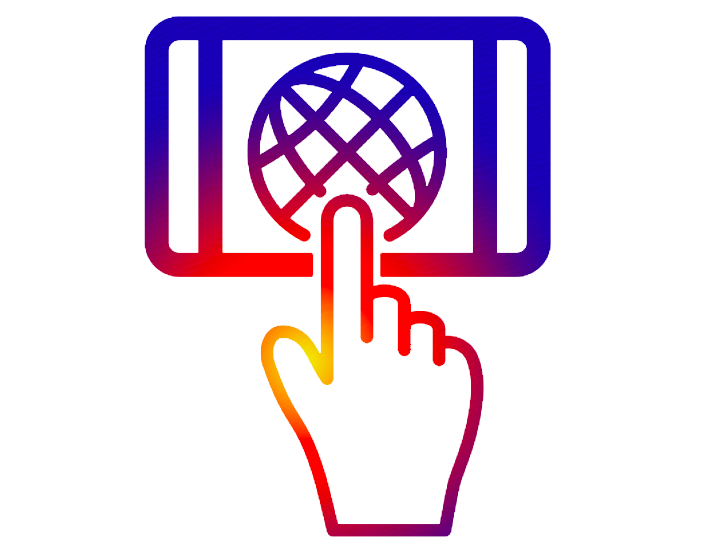
NOTE: The small spheres in the image above simply indicate the directions of maximum electron density. The shapes of the 2nd shell hybrid orbitals themselves will be more like three equal longitudinal sections that fill the volume within their shell with electron density. It will be highest at the center of the face of each orbital (as in the traditional sp2 lobe shapes) and will decrease toward the nodal regions between orbitals — as wave structures usually do — where electron density will be lowest (though not zero).
NOTE ALSO: Even though it is often useful to talk about these orbitals as separate, they are all — the entire atom is — part of a single, coherent, harmonic, resonant, phase-locked, spherically-symmetrical quantum wave state, and it is all electromagnetic at the root-energy level. Orbitals and their ‘boundaries’ can be seen as nothing more than nodes and antinodes in this harmonic wave structure.
The diagrams below show only boron’s three 3rd shell sp2-hybrid orbitals. Each of these three hybrid orbitals contains one electron:
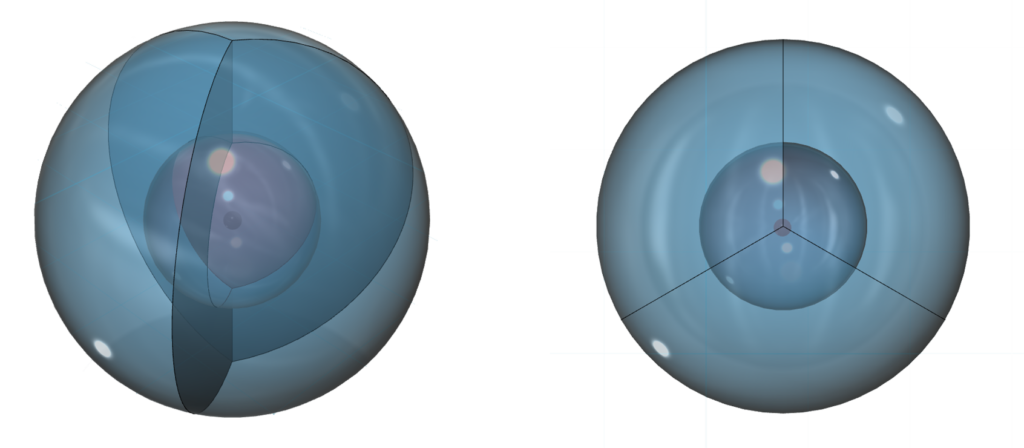
This arrangement is symmetrical in the equatorial plane (in 2 dimensions) but does not have equivalent symmetry in all directions. This makes boron keen to connect with more electrons in search of a 4th direction and spherical (tetrahedral) symmetry, like carbon. Four directions is more stable around a sphere than three directions.
Bonding & ion formation
Boron is therefore a versatile atom. It can bond ionically by losing its 3 valence electrons and forming the spherically symmetrical B3+ ion, but it will usually seek to gain electrons through covalent bonding. This duality underscores boron’s metalloid (semi-metal) character, as well as its versatility in that it can bond in either 3 directions by pairing its three unpaired electrons, or 4 directions by accepting two electrons from another atom. This form of covalent bonding, where another atom donates both electrons for the bond, allows boron to form adducts — combination molecules like B2H6 — and molecular structures like BH3NH3. It is also why boron could be doped into both trigonal and tetrahedral carbon crystals.
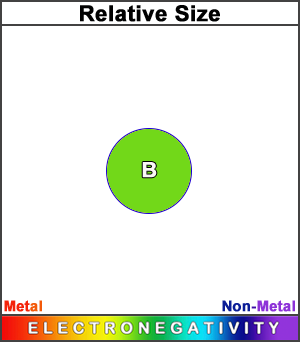
Boron has a smaller atomic radius than beryllium. (This square represents the size of the largest atom). The decrease in size across the period results from an increase in effective nuclear charge.
Its electronegativity value is too high to be a metal, but too low to be a non-metal. This is what makes it a semi-metal (or metalloid).
Uses
Boron is small and makes multiple covalent bonds. It is used as an additive in fiberglass, glass, and also in the manufacture of materials that need to be very hard or strong and light-weight, such as boron carbide ceramics.
When boron atoms replace carbon atoms in a carbon crystal, they create points of lower electron density (or relative positivity) in the crystal because boron atoms contain 5 electrons while the carbon atoms surrounding them each have 6 electrons. (If nitrogen were doped into an adjacent region of the crystal, it would have points of relative negativity because nitrogen atoms contain 7 electrons.) Such doping gives a crystal important properties, which are leveraged, for example, when making a p–n junction diode, used in electronics and photovoltaic (solar) cells.
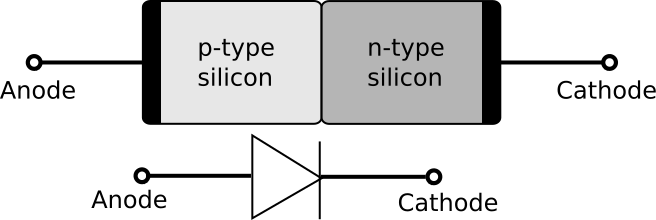
RETURN to the Periodic Table