
RETURN to Periodic Table
Carbon is the 6th element on the periodic table because it has 6 protons in its nucleus. The protons will attract 6 electrons to surround the nucleus in order to form a neutral atom. With 6 protons and 6 neutrons, most carbon atoms have an atomic mass of 12 amu.
Electron Shell
Carbon has two electrons in its p-orbital. These are not sphere-shaped harmonics, and like boron, carbon’s p-electrons cannot achieve symmetry around a sphere on their own because they lie in orthogonal directions.
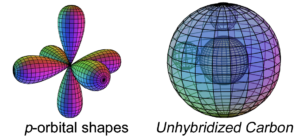
Carbon therefore cannot simply add its two p-electrons on top of the same (2s2) configuration that beryllium has, as shown above, because it would not be a stable configuration. The asymmetry therefore causes the two electrons in the 2s orbital to uncouple from their di-electron state and hybridize with the two p-electrons in order to achieve 4-directional, tetrahedral symmetry.
Electron Orbital Hybridization
Through hybridization, the four 2nd shell electrons now have equal energy (degenerate) and they can achieve maximum stability by assuming a tetrahedral arrangement around the core electron shell. Carbon is therefore the first atom that can achieve this 4-directional electron geometry on its own, which is why carbon typically makes 4 bonds. (The large wireframe below indicates the boundary of the n=2 shell.)

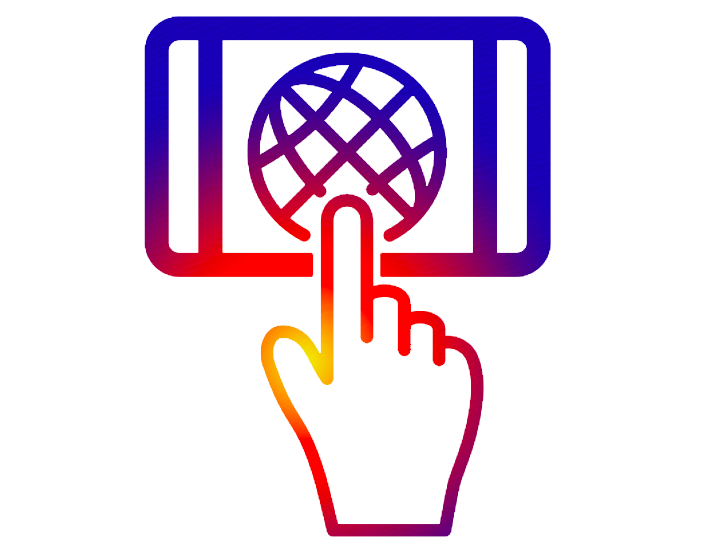
NOTE: The small spheres in the image above simply indicate the directions of maximum electron density. The shapes of the 2nd shell hybrid orbitals themselves will be more like spherical tetrahedra that fill the volume within their shell with electron density. It will be highest at the center of the face of each orbital (as in the traditional sp3 lobe shapes) and will decrease toward the nodal regions between orbitals — as wave structures usually do — where electron density will be lowest (though not zero).
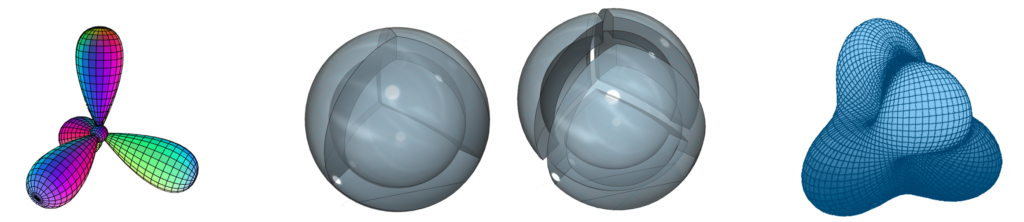
NOTE ALSO: Even though it is often useful to talk about these orbitals as separate, they are all — the entire atom is — part of a single, coherent, harmonic, resonant, phase-locked, spherically-symmetrical quantum wave state, and it is all electromagnetic at the root-energy level. Orbitals and their ‘boundaries’ can be seen as nothing more than nodes and antinodes in this harmonic wave structure.
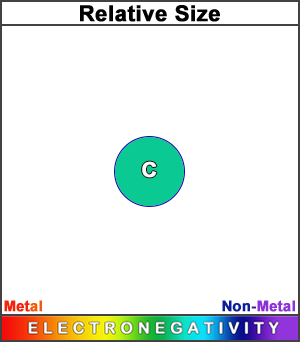
Carbon has a smaller atomic radius than boron. (This square represents the size of the largest atom).
Both its decrease in size and its increase in electronegativity (across the period) result from its larger effective nuclear charge. Its resulting unwillingness to give up an electron makes it non-metallic, causing carbon to favor covalent bonds.
Bonding & ion formation
While carbon can form the carbide (C4–) ion, it typically bonds covalently.
Carbons small size, being only the 6th element, as well as the fact that it can bond in up to four directions, makes it one of the most versatile elements, structurally. It can facilitate linear, trigonal, or tetrahedral electron geometry and it can make single, double, triple, or resonance bonds in linear, bent, or ring configurations.
Carbon can form structures as hard and beautiful as diamond, as strong as carbon fiber, as fine and versatile as graphene and nanotubes, as well as being the spindle around which the chemistry of life itself turns. It is almost single-handedly responsible for organic chemistry, which makes genetic information storage and biochemistry possible.
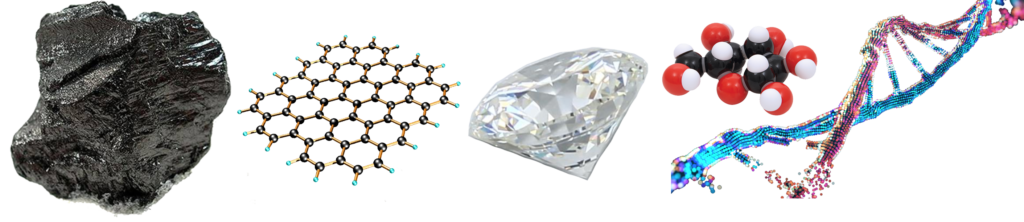
Methane (CH4)
When carbon bonds covalently with 4 hydrogen atoms, the symmetrical and non-polar methane (natural gas) molecule is formed.
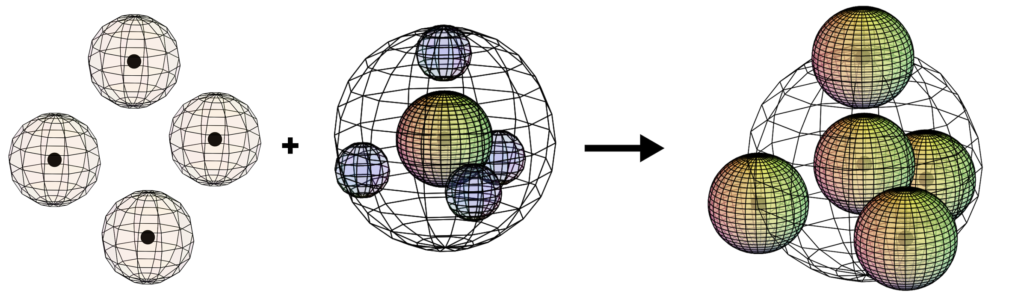
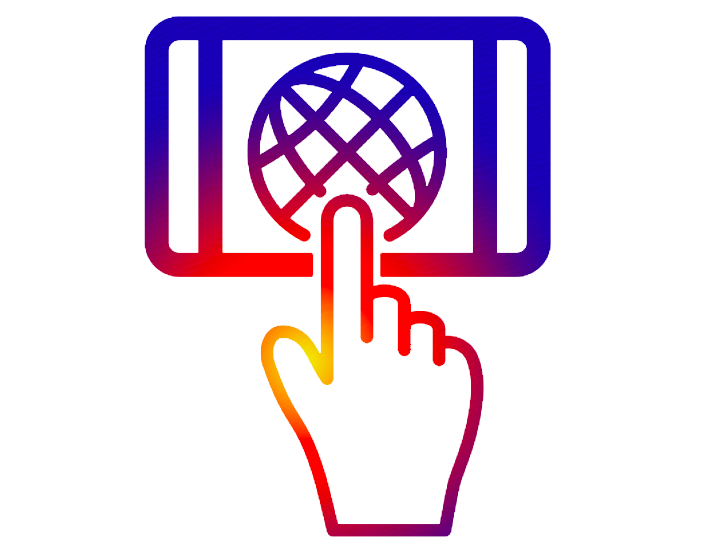
Carbon in Nature
Methane (CH4) can be “oxidized” in air. A little instigating energy will cause molecular bonds to be destabilized just enough to allow the oxygen in the air to attack both the hydrogen and the carbon in the methane molecule. Since carbon and hydrogen both have a lower electronegativity than oxygen, they provide an easier source of electrons for oxygen atoms than oxygen atoms provide for each other. The result is that oxygen atoms from the oxygen molecules (O2) in the air let go of one another and instead bond to all of the carbon and hydrogen atoms in the methane. Water vapor (H2O) and carbon dioxide (CO2) are formed as a result. This is an example of a combustion (burning) reaction. The chemical equation for this reaction is:
CH4 + 2O2 —> 2H2O + CO2
Interestingly, it is then these two products (H2O & CO2) that plants take in and convert into sugar (C6H12O6) and oxygen (O2) through photosynthesis. As such, the more trees and green plants on the planet, the more carbon dioxide (CO2) is removed from the atmosphere. The more phytoplankton and kelp forests in the ocean, the more carbon dioxide (CO2) is removed from the water, and thereby indirectly from the atmosphere.
Carbon dioxide is a greenhouse gas, which means that its presence in the atmosphere plays a role in the amount of heat absorbed by the atmosphere. Many seek to limit its presence in the air not only by decreasing the combustion of fossil fuels but also using technologies that remove carbon dioxide from the atmosphere. As these carbon sequestration technologies are investigated, we should note that photosynthesis is the most effective carbon sequestration technology at hand. It lowers atmospheric carbon levels and provides valuable nutrients to the ecosystem.
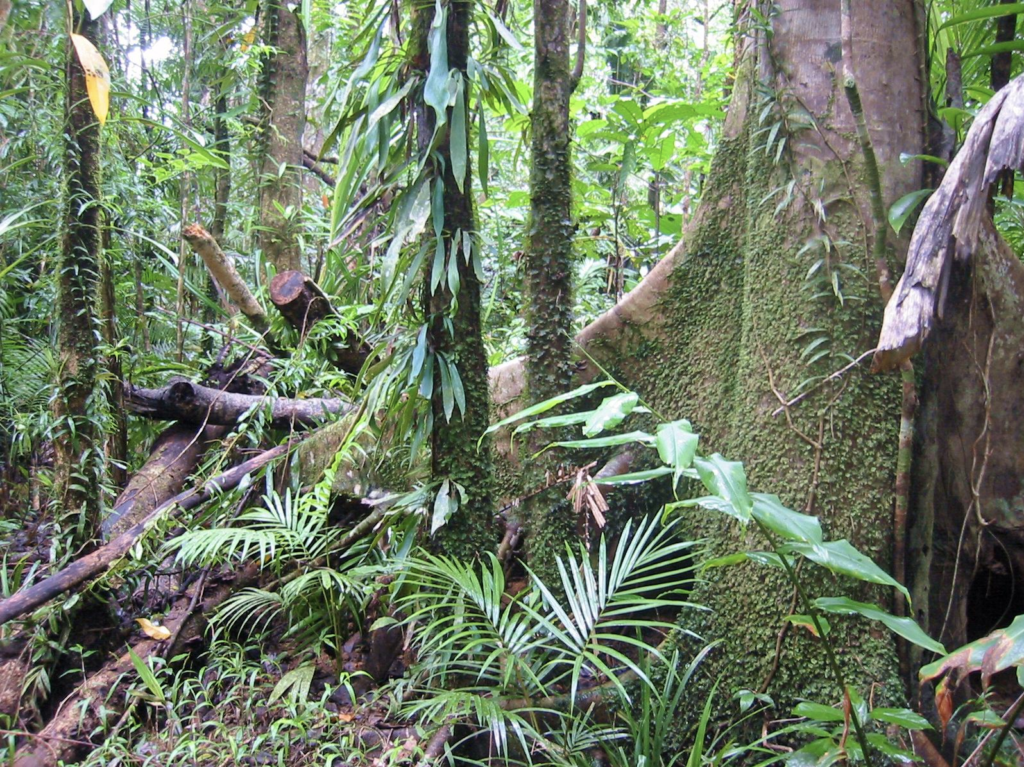
If we fight to limit carbon emission but we do not stop global deforestation, our gain is offset by our loss, and our climate problems will worsen. Not to mention the rate of species extinctions, each of which plays a valuable role in trapping carbon into the soil and life cycle.
RETURN to the Periodic Table